Multiphoton Microscopy
Authors: Maura Francis, Cory Boone, Jaclyn Wycoff
Multiphoton, or non-linear, microscopy, is ideal for capturing high-resolution three-dimensional (3D) images with reduced photobleaching and phototoxicity compared to traditional confocal microscopy techniques. This is the preferred method for analyzing thick samples of living tissue because of the technique’s advanced optical sectioning without out-of-focus absorption.
Multiphoton microscopy is a subset of fluorescence microscopy that uses similar imaging techniques of conventional widefield fluorescence microscopy to differentiate structures in living cells or tissues with various fluorescent tags known as fluorophores. Laser wavelengths of sufficient energy will excite the fluorophores to emit light that generates an image. Only one photon is needed to excite a fluorophore in conventional techniques. Out-of-focus fluorescence is often an issue in standard fluorescence microscopy due to fluorescence from other planes interfering with the focal plane. Traditional confocal microscopy combats this issue by blocking any out-of-focus light with a pinhole in front of the detector. However, this technique is not ideal for imaging thick specimens because of scattering and absorption. The image quality starts to deteriorate at thicknesses of around 100µm, but in certain samples image quality can be maintained as far as 700µm.1
As its name implies, multiple photons are absorbed simultaneously to excite fluorophores in multiphoton microscopy. The excitation light is confined to a very small three-dimensional volume (Figure 1), so this technique experiences lower levels of scatter compared to traditional confocal microscopy. The amount of light scattered is also inversely proportional to the fourth power of the light’s wavelength. Traditional techniques use ultraviolet (UV) or visible lights, which scatter considerably more than infrared light. The reduced scatter and absorption of the infrared wavelengths typically used in multiphoton microscopy allow for deeper penetration within a sample.2
Figure 1: The excitation light resulting from multiphoton microscopy is confined to a much smaller region of the sample than that resulting from single photon techniques.
Multiphoton and confocal microscopy are almost identical in terms of instrumentation, but multiphoton microscopy uses ultrafast lasers, such as femtosecond lasers. Ultrafast lasers have high peak power and short pulse duration, both of which are needed to excite the fluorophores. Many of these lasers emit in the NIR to IR regions and have high repetition rates, which has shown to be beneficial in biological, living samples.3 The high peaks in power allow for a higher resolution in imaging and contribute to the reduction in scattering. Unlike confocal microscopy, multiphoton microscopy does not require a pinhole because the out-of-focus fluorescence is significantly reduced due to the three-dimensionally confined excitation volume.
How It Works
A window is sometimes implanted into the subject being examined for maximum tissue penetration. Light penetration is typically limited to 1-2mm through the tissue itself. By using an infrared (IR) laser such as a Ti:Sapphire laser, less light is scattered and penetration depth increases.4 Longer wavelength photons have less energy than shorter wavelength photons and so, multiple IR photons are then needed to excite one fluorophore to a higher energy state (Figure 2). If a fluorophore typically gets excited with a photon wavelength of 350nm, then in two-photon microscopy that same fluorophore can get excited by two photons each with a wavelength of 700nm. The same concept applies to three-photon microscopy, except that three photons with triple the wavelength required for single-photon absorption are needed to excite the fluorophore. For multiple photons to contribute to a single excitation, the time lapse between them needs to be extremely short. This is why ultrafast lasers with short pulse durations and high repetition rates are needed.
Additional benefits of using the NIR wavelengths are that they allow for repetitive imaging and less scatter than UV wavelengths, which may also cause extensive photobleaching. Multiphoton microscopy circumvents several of the drawbacks of techniques based around UV excitation and allows users to image further into a specimen.4 UV wavelengths also cause more photodamage in biological samples.
Figure 2: In single-photon absorption (A), a single ultraviolet (UV) photon is absorbed to produce fluorescence. Multiphoton microscopy utilizes either two-photon (B) or three-photon (C) absorption, in which multiple photons are absorbed to produce fluorescence.
Multiphoton excitation will occur when the energies of multiple photons incident at the fluorophore simultaneously match the transition energy required to excite it from its ground state. The fluorophore will absorb two or three photons at once, depending on the technique (two- or three-photon microscopy). Absorption depends on the square of the intensity of excitation, so two- or three-photon absorption will only occur at the system’s focal point, where the photon density is the highest. This prevents any out-of-focus fluorescence from obscuring the image and eliminates the need for a pinhole, differentiating multiphoton microscopy from traditional confocal microscopy. Quick continuous pulses are sent into the system to increase the probability of simultaneous absorption.2 When such a laser is focused, the photons become more crowded, thus spatial density will increase.4 This also will increase the overall probability of fluorophores interacting at the same instant. Laser pulses with high peak powers and pulse durations on the order of femtoseconds are required for multiphoton excitation.
Image Appearance
Since all fluorescence comes from a small volume at the point of focus, multiphoton microscopy collects the intensity of all the emitted light that comes from the specimen.5 The excitation laser is scanned across the sample to trigger fluorescence and generate the full field of view of the image. Because multiphoton absorption does not occur outside the focal volume, high-resolution images at different depths in the sample can be obtained. Due to the localized excitation causing no out-of-focus light to obscure the image, two-photon microscopy has the same resolution in the x- and y-axes as traditional confocal microscopy.5 Two- and three-photon microscopy techniques have a better z-axis resolution due to the fact that out-of-focus fluorescence is minimized in three-dimensions.5 This causes smaller cross-sectional areas to be collected, allowing for finer details to be distinguished, as seen in Figure 3.
In most configurations of multiphoton microscopy systems, photomultiplier tubes are used to detect signals with high sensitivity. This setup allows for fast data acquisition, which would be beneficial in applications that require near instantaneous feedback. Machine vision cameras with either charged couple device (CCD) or complementary metal-oxide semiconductor (CMOS) detectors can also be used in additional pathways to acquire a widefield image of the sample.2

Figure 3: An image of a mouse tumor captured using multiphoton microscopy, courtesy of the Laboratory for Optical and Computational Instrumentation (LOCI) at the University of Wisconsin, Madison.6
Technical Details
Figure 4 depicts the typical layout of a multiphoton microscope. A multitude of optics are present in a multiphoton system. The typical components that make up a multiphoton system are:
- IR Laser Excitation Source: IR source, such as a Ti:Sapphire or ultrafast fiber laser, emits a laser beam with femtosecond pulse durations and a high enough peak irradiance to induce multiphoton absorption in the sample. The high cost of Ti:sapphire lasers previously made this technique cost-prohibitive for certain situations, but newer and less expensive fiber laser sources now make multiphoton microscopy more accessible.
- Low group delay dispersion (GDD) Mirrors: their low GDD helps minimize chromatic dispersion and pulse spreading. These mirrors feature dielectric coatings optimized to provide high reflectance for the laser wavelength for which they are designed and to minimize GDD. Dichroic low GDD mirrors are used to separate emission signals at different fluorescence wavelengths, while low GDD scanning mirrors are used to pass emitted fluorescence to a detector.
- Bandpass, shortpass, longpass, and dichroic filters: different types of filters, typically bandpass or shortpass, are used to clean up excitation laser wavelengths and then to select particular wavelengths for detection.7 The filters allow for the nonlinear signals to be passed. Dichroic filters are often used to separate excitation and emission wavelengths.
- Objective: often a water or oil immersion infinity corrected objective, this component focuses the excitation source onto the sample, inducing multiphoton absorption at the focal point. Fluorescence is then collimated back through the objective and passed along to the detector. Objectives for multiphoton microscopy typically have a high numerical aperture (NA), high magnification, chromatic correction over a wide waveband, and a long working distance.
- Tube lens: placed behind the objective to focus the collimated fluorescence to obtain a primary image.
- Detector: a photomultiplier tube (PMT), imaging lens and camera, or other detector setup collects the fluorescence emitted from the focal plane, resulting in high-resolution images. 3D images can be generated by scanning through different planes of focus and stitching the images together.

Figure 4: Typical schematic of a multiphoton microscopy system.
Applications of Multiphoton Microscopy
Application 1: Brain Imaging
Multiphoton microscopy has allowed researchers to image deep into brain tissue in a way that they have not been able to do before. By employing a three-photon technique, researchers are able to image down into the hippocampus, which provides insight into how neurons are generated in the brain.8 Scientists are able to image in vivo, which can be extremely beneficial for diagnosing different pathologies. A study performed in mice employed multiphoton imaging to explore the functional effects of neurons and astrocytes in the progression of Alzheimer’s disease. Using in vivo imaging to see these structures can give scientists a greater understanding to the pathogenesis of the disease. By being able to see the brain via multiphoton microscopy, scientists and researchers can delve into how the disease progresses and develop better methods for treatment and diagnosis.9
Application 2: Retina Imaging
Multiphoton microscopy has also been successful in imaging the retina of the eye. Traditional methods of retina imaging prevent the observation of subcellular structures due to the optical aberrations the eye presents.7 Retina imaging can diagnose certain eye diseases and also identify degenerative brain diseases because of the retina’s role in the central nervous system. This can provide clues to onsets of diseases like Alzheimer’s or multiple sclerosis. Using multiphoton microscopy is especially beneficial in imaging the eye because the NIR lasers do not interfere with visible stimulation and the eye is optically transparent to longer wavelengths.7 The high spatial density of multiphoton microscopy provides excellent capabilities to evaluate the structures within the eye and gain insights into disease mechanisms.
Comparison to Other Microscopy Techniques6
Technique | Resolution | Sample Size | Relative Cost | Photobleacing |
---|---|---|---|---|
Confocal Microscopy | <µm | µm | $$ | Yes |
Multiphoton Microscopy | <µm | mm | $$$ | Less |
Light Sheet Fluorescence Microscopy | µm | >cm | $ | Least |
Multiphoton Microscopy at Edmund Optics®
Edmund Optics® supplies a wide range of optics for multiphoton microscopy applications including low group delay dispersion (GDD) mirrors, filters, objectives, polarizers, and gratings. More components ideal for these systems are continuously added as the multiphoton microscopy application space continues to grow.
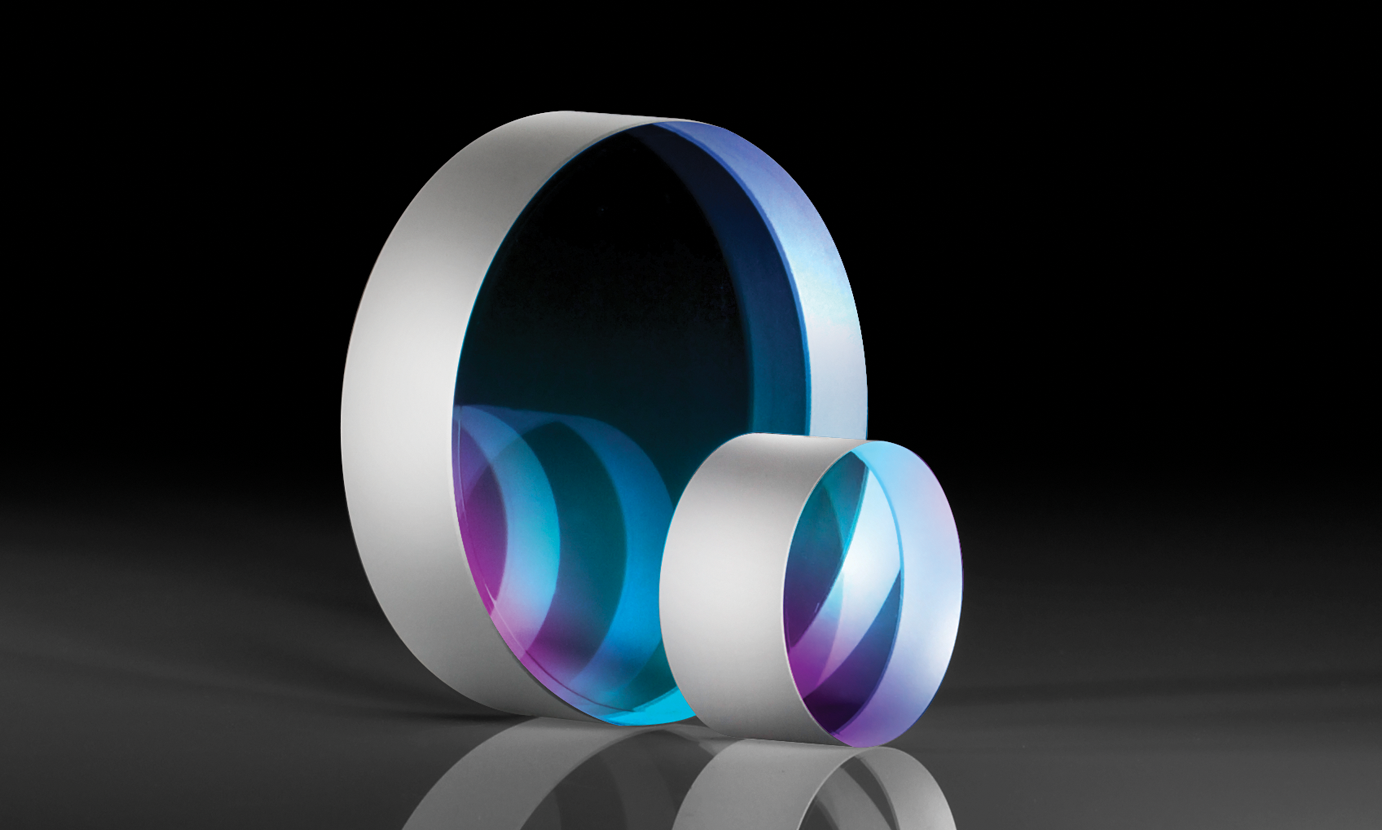
Low GDD Mirrors
- Designed with High Reflectivity for Ultrafast Beam Steering
- Ion-Beam Sputtered Coated Options for Low Scatter and Absorption
- High Laser Damage Threshold Options
- GDD Down to 0±20fs2 at Design Wavelength Range
BUY NOW

Ultrafast-Enhanced Silver Laser Mirrors
- Reflectivity >99% at 600 – 1000nm or 800 – 1150nm
- Low Group Delay Dispersion as Low as 0 ±20fs2
- Ideal for Ti:sapphire and Yb:doped Lasers
- Standard Imperial Sizes Available
BUY NOW
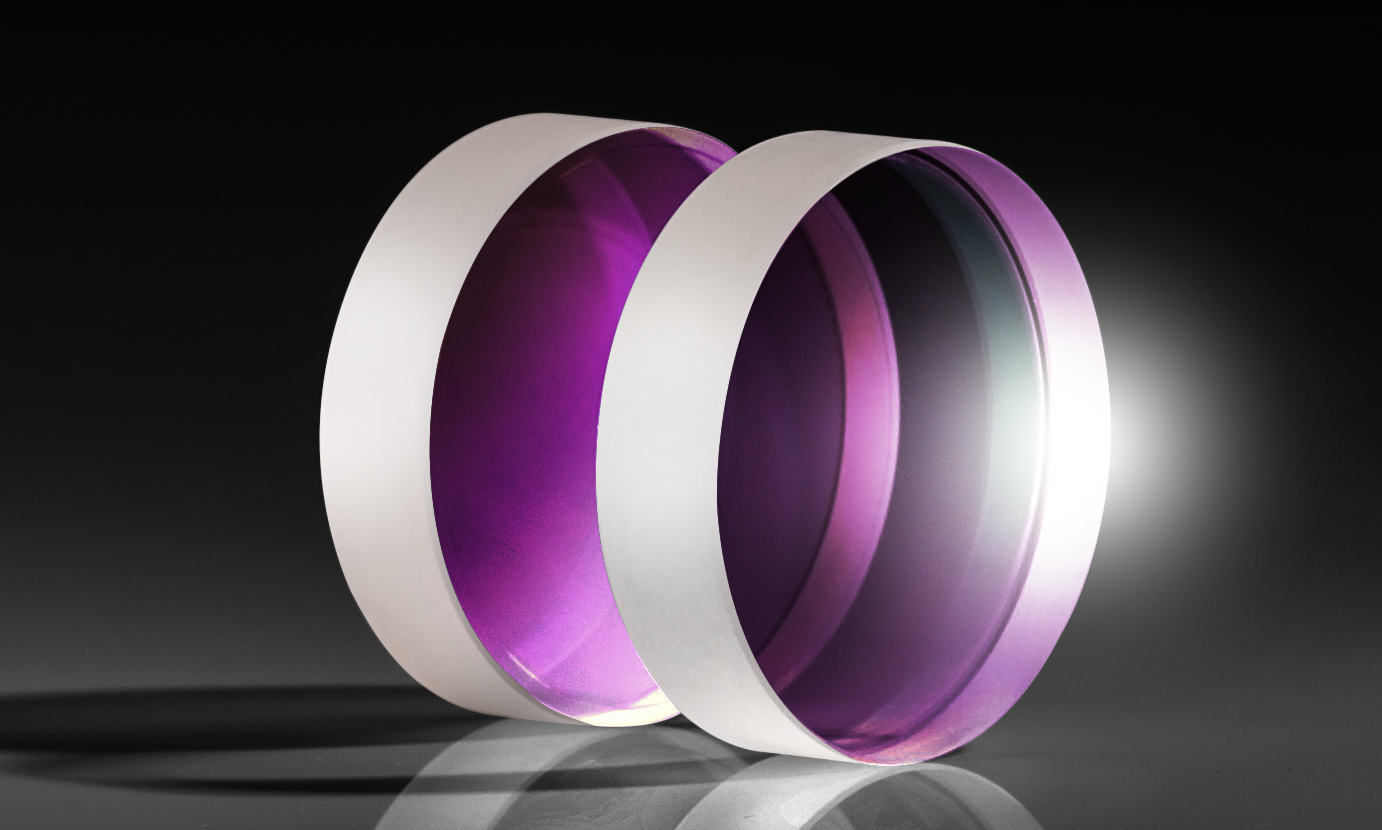
UltraFast Innovations Pulse Compression Mirrors
- Highly-Negative Group Delay Dispersion
- Ideal for Ultrafast Pulse Compression
- Variety of Coatings for Common Wavelengths
- Industry-Leading Laser Optics from UltraFast Innovations
BUY NOW

Low GDD Dichroic Shortpass Ultrafast Filters
- Reduced Thickness for Limited GDD
- Broad Transmission and Reflection Ranges
- Ideal for Ultrafast Laser Applications
- Controlled GDD Specification Using In-House Metrology
BUY NOW
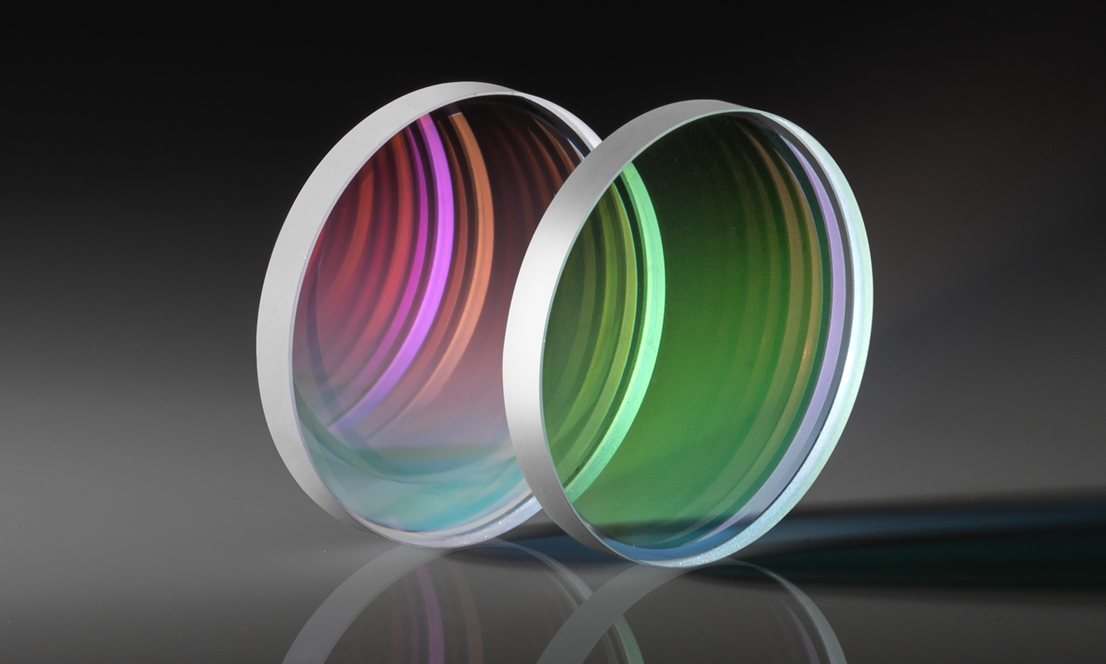
Ultrafast Thin Film Polarizers
- Ideal for Ti:sapphire and Yb:doped Ultrafast Lasers
- Optimized for Separation of S- and P-Polarizations at 45° AOI
- High Extinction Ratio of 1000:1 @ DWL
BUY NOW
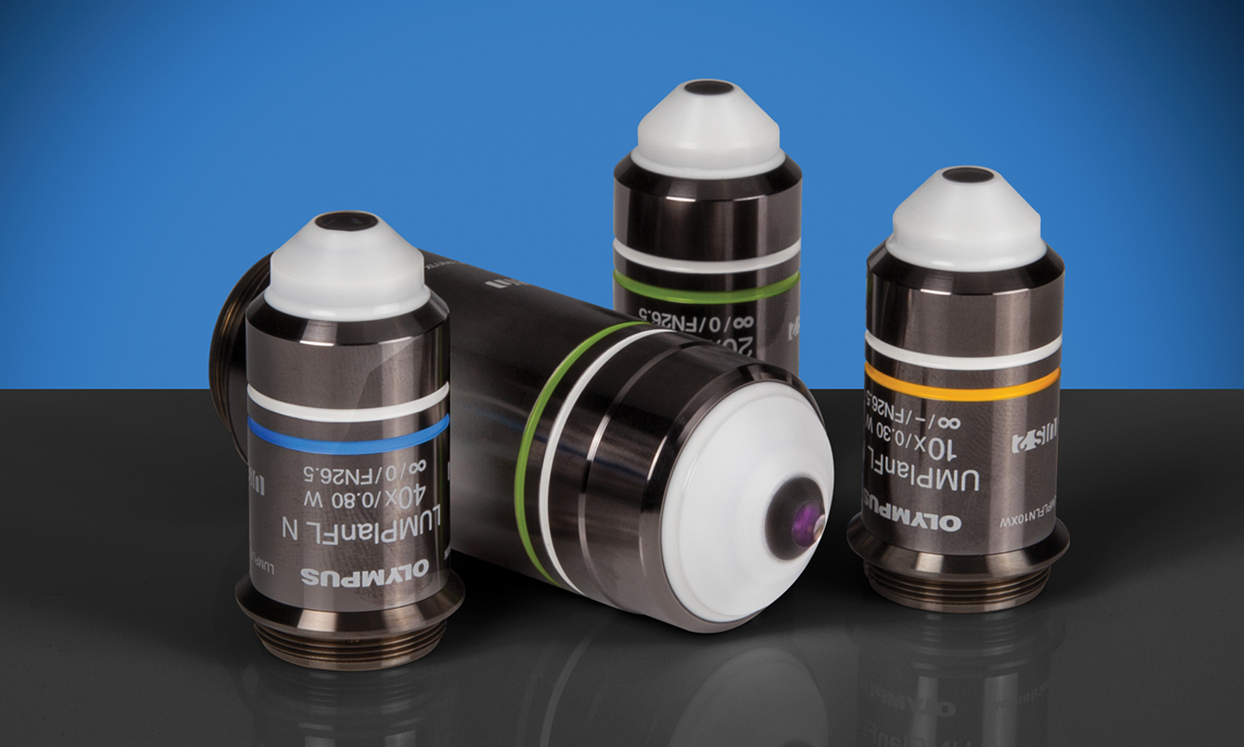
Olympus Water Immersion Objectives
- Water Immersion Objectives with Magnification Ranges from 10 to 40X
- Displays Flat Images from High Transmission Factors up to the Near-Infrared Region of the Spectrum
- Ideal for Fluorescence Imaging of Tissue and Specimens, such as Brain Tissue
BUY NOW

Olympus X-Line Extended Apochromat Objectives
- High Numerical Aperture (NA) up to 1.45
- Chromatic Aberration Correction from 400 - 1000nm
- Uniform Image Flatness over Large FOVs
BUY NOW

Mitutoyo Infinity Corrected Long Working Distance Objectives
- Long Working Distances
- Bright Field Inspection
- High Quality Plan Apochromat Design
- Flat Image Surface over Entire Field of View
BUY NOW

Reflective Objectives
- Long Working Distance for Simple System Integration
- Actively Aligned for Optimal Performance
- Ultra-Wide Spectral Band from 190nm to 11μm with No Chromatic Aberration
- Diffraction-Limited Options with Transmitted Wavefront of λ/14 RMS
BUY NOW

Diffraction Gratings
- Wide Variety of Ruled and Holographic Designs
- High-Precision Options with Groove Spacing Tolerance <0.05%
- Custom Sizes Available of Many Grating Types
BUY NOW
References
- Allen, John R. “Light Sheet Fluorescence Microscopy.” Nikon, https://www.microscopyu.com/techniques/light-sheet/light-sheet-fluorescence-microscopy
- Piston, David W., et al. “Multiphoton Microscopy.” Nikon, https://www.microscopyu.com/techniques/multi-photon/multiphoton-microscopy.
- “Two-Photon Microscopy.” Laser Quantum, https://www.laserquantum.com/applications/detail.cfm?id=24#:~:text=The%20laser%20used%20for%20two,interested%20in%20imaging%20living%20cells.
- Larson, Adam M. “Multiphoton Microscopy.” Nature, 22 Dec. 2010, https://www.nature.com/articles/nphoton.an.2010.2.
- Davidson, Michael W., and David W. Piston. “Introduction to Multiphoton Fluorescence Microscopy.” Olympus Scientific Solutions Americas Corp., https://www.olympus-lifescience.com/en/microscope-resource/primer/techniques/fluorescence/multiphoton/multiphotonintro/
- Wendt, Kristy. “Multiphoton Microscopy and Second Harmonic Generation.” Laboratory for Optical and Computational Instrumentation, University of Wisconsin, Madison, https://loci.wisc.edu/research/two-photon.
- Qin, Zhongya, et al. “Adaptive optics two-photon microscopy enables near-diffraction-limited and functional retinal imaging in vivo.” Light: Sciences Applications, https://www.nature.com/articles/s41377-020-0317-9
- May, Mike. “Shedding light on deep tissue: Multiphoton microscopy.” Science Magazine, https://www.sciencemag.org/features/2019/03/shedding-light-deep-tissue-multiphoton-microscopy.
- Kelly, Patricia et al. “In Vivo two photon imaging of astrocytic structures and function in Alzheimer’s disease.” Aging Neurosci, https://www.frontiersin.org/articles/10.3389/fnagi.2018.00219/full
- Santi, P. A. (2011). Light Sheet Fluorescence Microscopy. J Histochem Cytochem, 59(2), 129-138. doi: 10.1369/0022155410394857.
Additional Resources
- Brightfield Illumination Microscopy
- Darkfield Illumination Microscopy
- Confocal Microscopy
- Differential Interference Contrast Microscopy
- Fluorescence Microscopy
- Light Sheet Microscopy
- Phase Contrast Microscopy
- Digital Microscope Objective Setups
- Understanding Microscopes and Objectives
- Ultrafast Dispersion
- Ultrafast Optics: Challenges and Solutions
- Highly-Dispersive Mirrors
- Ultrafast Lasers – The Basic Principles of Ultrafast Coherence
or view regional numbers
QUOTE TOOL
enter stock numbers to begin
Copyright 2023, Edmund Optics Inc., 101 East Gloucester Pike, Barrington, NJ 08007-1380 USA
California Consumer Privacy Act (CCPA): Do Not Sell or Share My Personal Information
California Transparency in Supply Chains Act